In this video, hear from researchers Jeunghoon Lee and Olya Mass about the exciting work being done in Boise State’s quantum research program. This video is available with captions and a transcript.
Exploring Quantum Research at Boise State
Imagine you are holding a glass paperweight in your hand. Now use your eyes to zoom in, closer, closer. Then zoom in to depths beyond the abilities of any eyeball until you can see the space between the atoms of the paperweight. Welcome to the quantum world. At this spatial scale, we note that matter and energy are interconnected. Deep, right?
At Boise State, a highly specialized team of over 30 students, faculty and staff are part of the Quantum DNA Research Group, known as qDNA. They are taking quantum research to a jaw-dropping new level. Even the U.S. Department of Energy and the Office of Naval Research think so. That’s why qDNA has received a cumulative total of more than $14 million dollars between 2019 and 2022.
Boise State is advancing the world’s scientific understanding of all that quantum phenomena promise: advancing light harvesting, biosensing and imaging, quantum computing and quantum entanglement.
Researchers are finding a way to study quantum phenomena at room temperature. You might say, “So, what?” To put it into perspective, current quantum computing methods require a temperature of 0.02 Kelvin (-459.7 degrees Fahrenheit). Outer space is 3 Kelvin. You know what your power bill looks like on a hot summer day while trying to keep your home cool? Just imagine the power bill and the energy consumption needed to bring your space to -459.7 degrees Fahrenheit. Room temperature for quantum applications is a big deal.
Let’s take a closer look at how this works, and more importantly, why it should matter to you.
Into the Labs
Let’s go from one lab to another and peek in at what’s going on. Keep in mind, Boise State’s qDNA teams are unlike most. Instead of being made up solely of tenured and non-tenured faculty, many teams are led by highly experienced professional research staff who focus predominantly on research, and mentoring students and early career staff.
The Dye Synthesis Team, led by Olya Mass, senior research scholar, develops custom-made dyes used in experiments. These dyes are important because without them, it would be impossible to create the desired phenomena at the quantum level (fun fact: not all molecules have color). And, these are not just any dyes. They are formulated to interact with light and transfer energy in the same way that chlorophylls do in plants.
“We take inspiration from photosynthesis to design our handmade systems for quantum computing,” Mass said. “The photosynthetic function is similar to how we want our quantum systems to function.”
To help discover which dye candidates will perform best for desired applications, the Theory and Simulation Team, led by Associate Professor Lan Li and Distinguished Research Fellow
Bernard Yurke, develops and implements computational modeling and machine learning techniques. The team also determines dye structure-property relationships and predicts how dyes interact with DNA scaffolds.
Next stop: the DNA Construct Synthesis Team led by Associate Professor Jeunghoon Lee.
“Our goal is to understand how energy is being transferred between molecules with as little energy loss as possible. Photosynthetic organisms have figured this out. When energy or light is being transferred from one molecule to another, we want to study and understand how that transfer can take place with the quantum information preserved between the molecules,” Lee said.
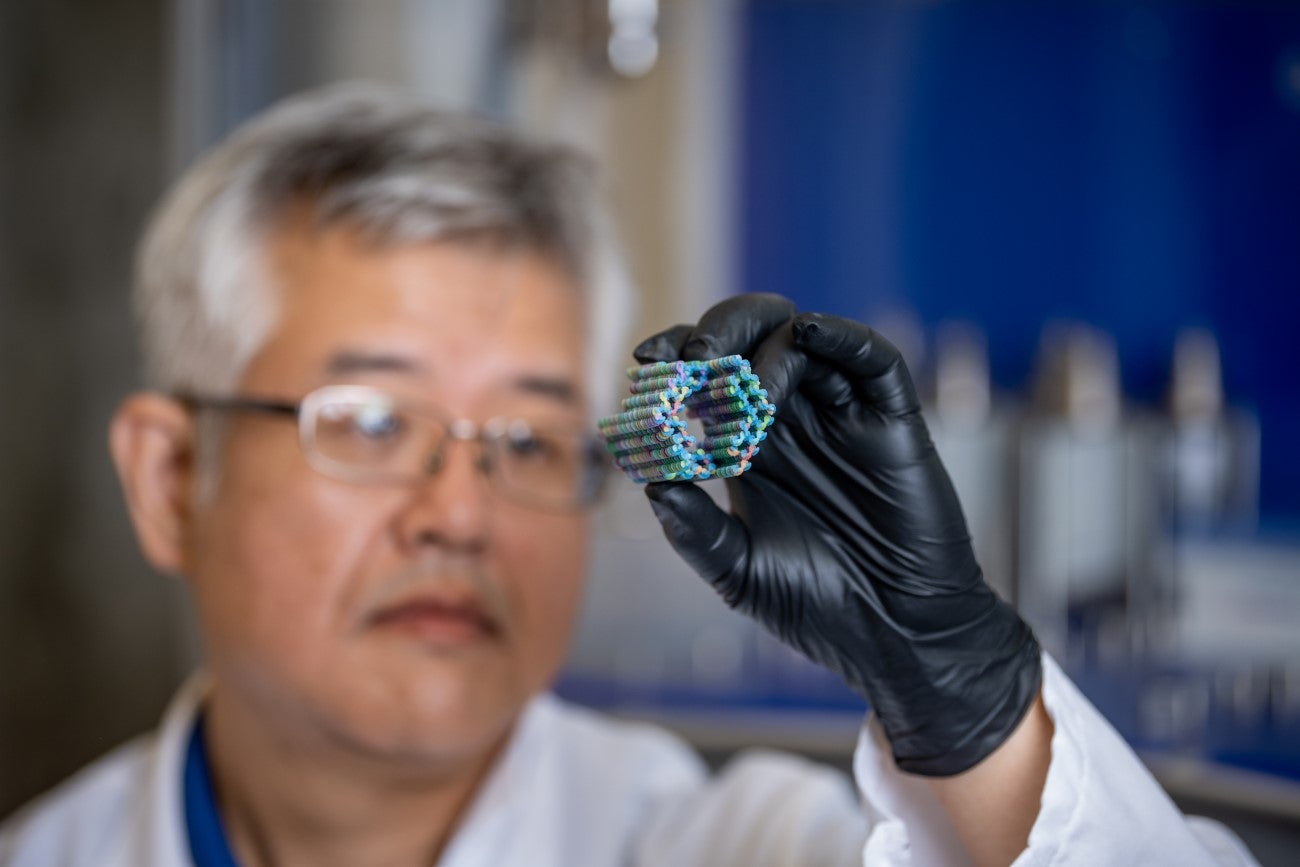
To do that, the team uses synthetic DNA. Unlike in the human genome, where the combinations of the four base pairs are used to store information in DNA, qDNA uses DNA as a molecular scaffold. In this case, to study how the quantum information is being preserved and manipulated between dye molecules, Lee and his team use these four base pairs like an extremely tiny, nano-
scale “Lego” set to organize the aforementioned dyes into desired frameworks, or dye aggregates.
Now, it’s time to bring in the lasers. The next step in this process introduces us to the UltrafastSpectroscopy Team, led by Principal Research Scholar Ryan Pensack, Lab Engineer Paul Davis and Principal Research Scholar Daniel Turner.
Although the team name sounds intimidating, Pensack brings us back to the literal meaning of the words to provide illumination: Ultrafast Spectroscopy (study of “ghosts” or “things unseen”).
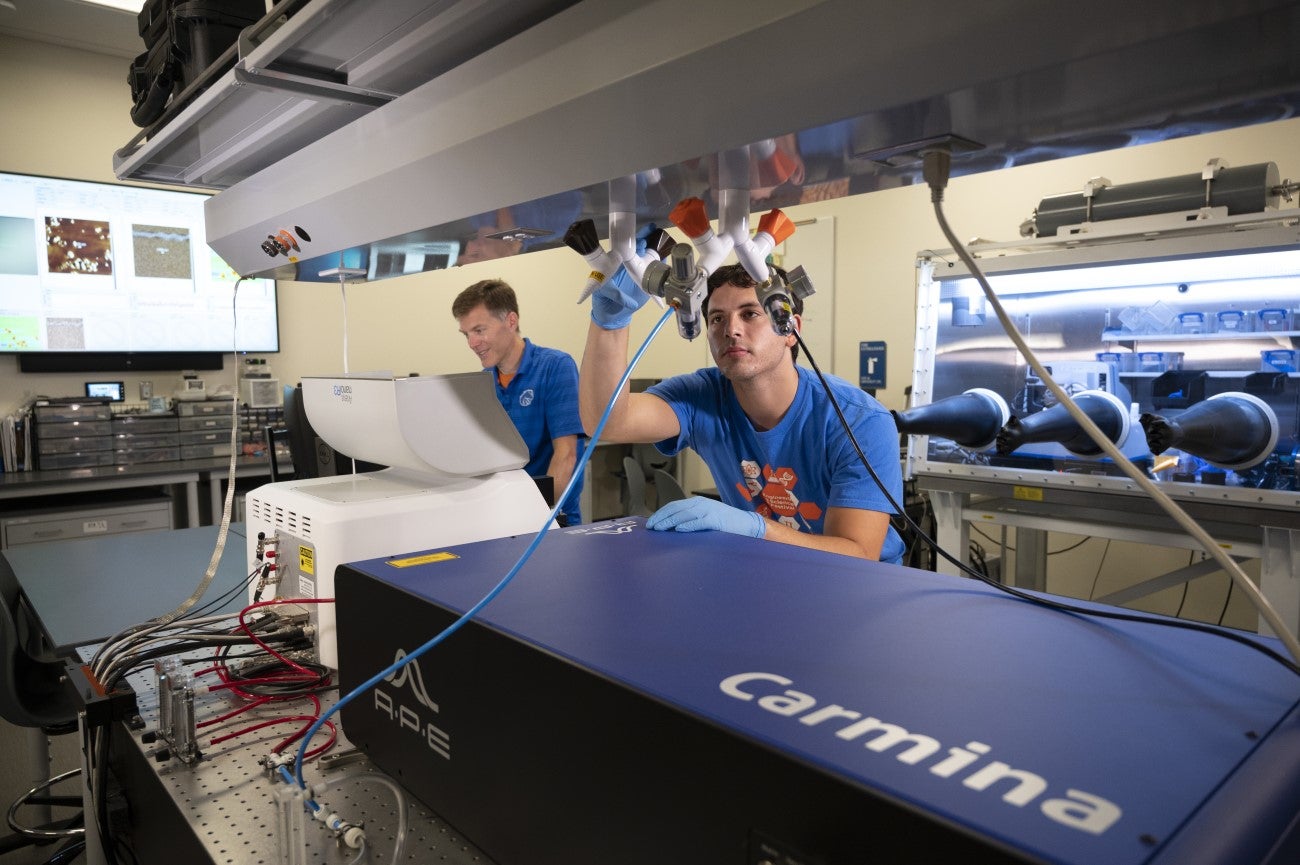
Pensack and his team use lasers that shoot beams of light at the DNA-assembled framework in pico- (one trillionth) and femto- (one quadrillionth) second bursts.
You might wonder, “Why?” Pensack reaches into his pocket for a spare quarter and spins it on his desk. Notice that when spinning, the quarter is no longer heads, or tails. It is both… and neither. This example, which Pensack attributes to IBM researcher Talia Gershon, is a visual example of the difference between a binary computer’s method of processing (0s and 1s) and a quantum computer’s processing potential.
By using lasers, the team excites the DNA dye aggregate and collects data to understand how long, and how frequently, the dyes exchange energy. These measurements are accessible with one-of-a-kind instrumentation thanks to Turner’s decades of experience in generating ultrashort laser pulses. With this data the team learns about spinning quantum states of the DNA-templated dye aggregate framework and how the environment interacts with these sensitive spinning quantum states (or superpositions).
The qDNA teams at Boise State bring the world closer to understanding quantum applications.
Everything these teams do together at Boise State brings the world closer to understanding how to make quantum applications feasible at room temp. Quantum information can be a key approach to testing all known possibilities and probabilities for problems such as vaccine efficacy, more sophisticated weather predictions, more accurate global positioning systems and financial forecasts.
That’s why the push to advance quantum computing is so important. It deals with probability and
potential outcomes in a way the human mind and current computers cannot.
—By Brianne Phillips, lab photos by John Kelly and Priscilla Grover, video by Matt Crook
Faculty and Professional Staff: Bill Knowlton, Bernie Yurke, Olya Mass, Jeunghoon Lee, Ryan Pensack, Daniel Turner, Paul Davis, Lan Li, Shibani Basu, Gissela Pascual Pariona, Matt Barclay, Katelyn Duncan, Keitel Cervantes-Salguero, Richard Elliott, Dipak Panthi, John Hall, Jennifer Edwards, Devan Watt, Lance Patten, Kimo Wilson, Madison Kadrmas, Lawrence Spear, Aaron Sup. Students: Simon Roy, Jon Huff, Nick Wright, Austin Biaggne, German Barcenas, Maia Ketteridge, Ryan Rau, Robbie Gill
Any opinions, findings, and conclusions or recommendations expressed in this article are those of the authors and do not necessarily reflect the views of the Department of Energy’s Office of Basic Energy Sciences, Materials Sciences and Engineering Division or Department of the Navy’s Office of Naval Research.
Support students and research in the College of Engineering.