Snow Distribution (WCWAVE Snow Camp Field Project)
Goal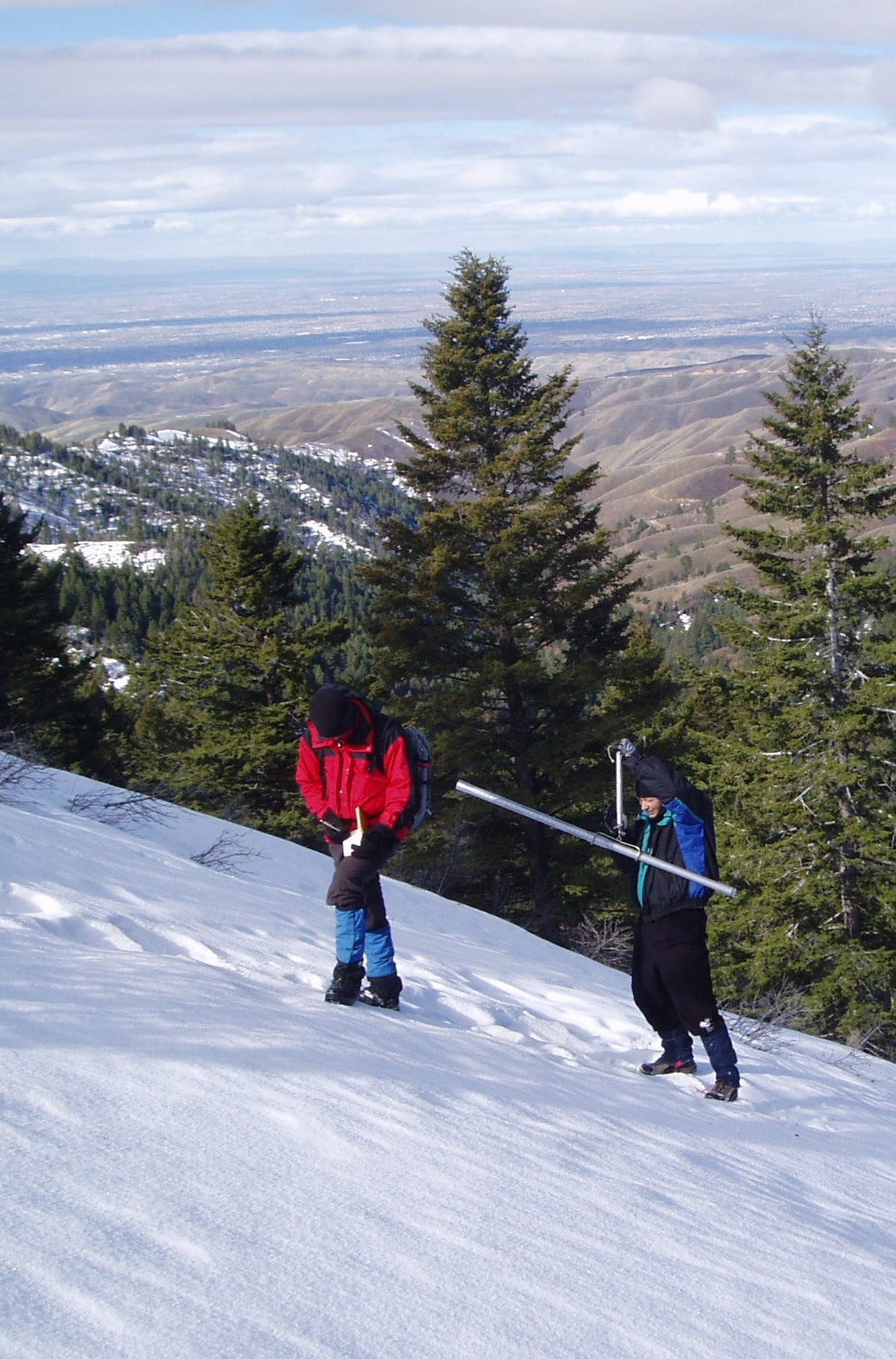
The goal of this project is to understand the role of snow accumulation and melt in the water balance of a mountain watershed, with a particular emphasis on how the spatial variability of snow impacts hydrologic response.
Approach
As a group, participants will design and implement a field campaign to estimate the volume of water stored as snow in the Dry Creek Experimental Watershed. Discussions and field demonstrations will focus on scales of variability from point to basin.
Learning Objectives
Upon completion of this exercise participants will be able to
- Make point measurements of snow depth, density and snow water equivalent (SWE)
- Describe relationships between terrain properties and snow distribution
- Design field campaigns to estimate the volume of water stored as snow in a watershed
- Describe processes controlling snow accumulation and melt in a mountain watershed
- Describe how spatially variable snow accumulation and melt impacts hydrologic response
Project Files
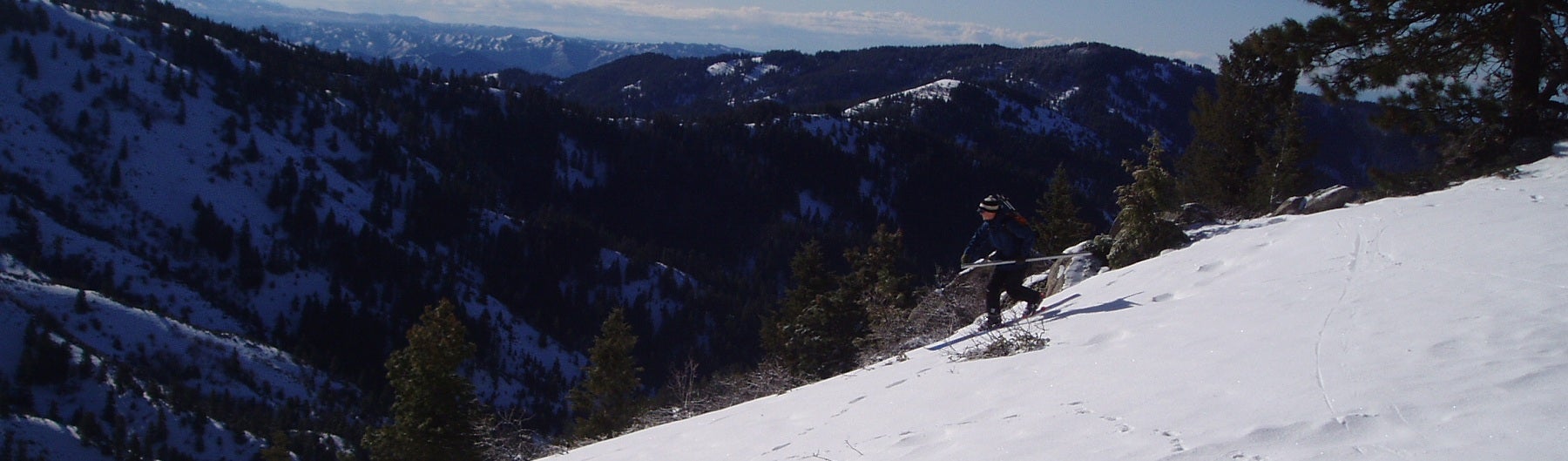
Quantifying the spatial and temporal distribution of water delivery to the land surface is an essential component of hydrologic modeling. In snow-dominated landscapes, however, water enters the land surface where and when snow melts rather than where and when it falls. Understanding the spatial variability of water stored as snow on the ground, called snow water equivalent (SWE), is therefore essential for predicting meltwater generation [Clark et al., 2011]. The distribution of SWE, however, is difficult to measure and can be substantially different than the distribution of snowfall measured by precipitation gauges [Scipión et al., 2013].
Empirical methods used to estimate where snow resides in mountain watersheds are commonly based on known correlations between SWE and terrain properties including elevation, aspect, slope, and canopy density [Anderton et al., 2004; Dixon et al., 2013; Elder et al., 1991; Elder et al., 1998; Jost et al., 2007; Molotch and Bales, 2005]. However, the underlying causes of correlations, which are often quantified from synoptic surveys at an assumed time of maximum accumulation, can be ambiguous. For example, correlation between elevation and SWE can be caused by orographic effects producing more precipitation at higher elevations [Houghton, 1979] and can also be caused by differential ablation or a transition from rain to snow with warmer temperatures at lower elevations [Jost et al., 2007; Tarboton et al., 2000]. Correlation between SWE and aspect is commonly explained by differential accumulation on lee and windward slopes [Harrison, 1986] but can also arise from differential ablation due to differences in solar loading on north versus south aspects [Clark et al., 2011; Meromy et al., 2012]. In areas of North America where dominant winds come from the southwest, the effects of wind and solar loading can be similar with less snow on windward, sunny slopes. An extensive body of literature recently reviewed by Lundquist et al. [2013] and Varhola et al. [2010] demonstrates that forest cover can enhance or inhibit both snow accumulation and melt depending on many interacting terrain and climate properties. Additionally, lateral flow of meltwater within the snowpack [Eiriksson et al., 2013], slope –induced gravitational transport [Kerr et al., 2013], and many other processes can further complicate SWE distribution by adding local variability with correlation lengths between 10-100 meters [Deems et al., 2008; Shook and Gray, 1998; Trujillo et al., 2007; Trujillo et al., 2009] to regional trends extending over several kilometers [Gillan et al., 2010].
The spatial distribution of SWE arises from season-long interplay between spatially and temporally heterogeneous snow accumulation and ablation processes [Tarboton et al., 2000]. Hence, the controls on snow distribution can change with time [Schirmer et al., 2011]. Clark et al. [2011] suggested that snow surveys conducted at an assumed time of maximum accumulation can bias conclusions concerning controls on snow distribution to favor accumulation processes. One-time synoptic snow surveys will reveal only information about the integrated processes responsible for the state of the snowpack up to that time. The potentially confounding relationships between observed correlations and underlying processes make if difficult, if not impossible, to ascribe causal relationships between basin-wide trends in SWE and particular processes. Process knowledge, however, is critical for evaluating the performance of snow accumulation and melt models [Blöschl et al., 1991]. Model improvement comes from understanding why models fail rather than just where they fail.[/one_half][one_half_last]
Regardless of the ambiguities surrounding the causes behind correlations between snow distribution and terrain properties, knowledge of such relationships can be used to design efficient field campaigns. A snow survey design to estimate SWE over an area should be extensive enough capture the spatial variability of SWE, yet be simple enough to be implemented field personnel within a limited amount of time. Areal estimation of mean SWE is accomplished in two general approaches including 1) index snow courses, and 2) spatial surveys.
Snow Courses such as those established by the Natural Resources Conservation Service (NRCS) rely on the notion that changes in mean SWE at an index station are equivalent to changes in SWE over a representative area. NRCS personnel visit stations routinely to measure SWE at the same location through a season. Over many years a relationship develops between small-scale mean SWE and larger scale water stored as snow. While snow courses are extremely valuable for many hydrology applications, they are of limited value for high resolution hydrologic modeling, particularly in basins with few or no established snow courses.
Spatial Surveys are used to measure “snapshots” of the distribution of SWE in an area of interest. Because of the large effort required to collect meaningful snow data, spatial surveys are typically only performed at an assumed time of maximum accumulation to provide estimates of the water available prior to the ablation season. Clearly, estimates of mean SWE in a basin improve with more measurements. Designing a spatial survey requires deciding 1) where to place measurement stations, and 2) what to measure at each station.
The distribution of measurement stations should be sufficient to capture large-scale sources of varability such as elevation, aspect, and canopy cover. Obtaining a statistically significant number of measurements over large area, however, is exceedingly difficult. Randomly selecting measurement locations is generally not possible because the number of measurements required to be statistically meaningful is generally prohibitive, and locations may be in inaccessible areas. Consequently, stratified sampling schemes are commonly employed. A stratified random sampling scheme discretizes an area of interest into regions that are expected to have similar values [Jost et al., 2007; Watson et al., 2006]. Points in the area of interest that share common relevant terrain properties including elevation, aspect, slope, and vegetation cover can be grouped by numerous geostatistical methods into representative snow units (RSU) (Figure 1). A field campaign can then be designed to cover examples of each RSU, rather than the entire area of interest.
The measurements taken at each station should be sufficient to capture small scale sources of variability such as wind patterns, local vegetation effects, and terrain roughness. Jost et al., [2007] used a cross pattern with two transects aligned parallel and perpendicular to aspect. Each transect consisted of 30 measurements of depth spaced 2 m apart. Snow density measurements were taken every 10 m. Other studies have used random distributions of depth and density within a diameter sufficient to overcome autocorrelation (references).[/one_half_last]
References
- Anderton, S. P., S. M. White, and B. Alvera (2004), Evaluation of spatial variability in snow water equivalent for a high mountain catchment, Hydrological Processes, 18(3), 435-453.
- Blöschl, G., R. Kirnbauer, and D. Gutknecht (1991), Distributed Snowmelt Simulations in an Alpine Catchment: 1. Model Evaluation on the Basis of Snow Cover Patterns, Water Resources Research, 27(12), 3171-3179.
- Clark, M. P., J. Hendrikx, A. G. Slater, D. Kavetski, B. Anderson, N. J. Cullen, T. Kerr, E. O. Hreinsson, and R. A. Woods (2011), Representing spatial variability of snow water equivalent in hydrologic and land-surface models: A review, Water Resources Research, 47, 23.
- Deems, J. S., S. R. Fassnacht, and K. J. Elder (2008), Interannual Consistency in Fractal Snow Depth Patterns at Two Colorado Mountain Sites, Journal of Hydrometeorology, 9(5), 977-988.
- Dixon, D., S. Boon, and U. Silins (2013), Watershed‐scale controls on snow accumulation in a small montane watershed, southwestern Alberta, Canada, Hydrological Processes.
- Eiriksson, D., M. Whitson, C. H. Luce, H. P. Marshall, J. Bradford, S. G. Benner, T. Black, H. Hetrick, and J. P. McNamara (2013), An evaluation of the hydrologic relevance of lateral flow in snow at hillslope and catchment scales, Hydrological Processes, 27(5), 640-654.
- Elder, K., J. Dozier, and J. Michaelsen (1991), Snow accumulation and distribution in an alpine watershed, Water Resources Research, 27(7), 1541-1552.
- Elder, K., W. Rosenthal, and R. E. Davis (1998), Estimating the spatial distribution of snow water equivalence in a montane watershed, Hydrological Processes, 12(1011), 1793-1808.
- Gillan, B. J., J. T. Harper, and J. N. Moore (2010), Timing of present and future snowmelt from high elevations in northwest Montana, Water Resources Research, 46(1), W01507.
- Harrison, W. (1986), Seasonal accumulation and loss of snow from a block mountain catchment in central Otago, Journal of Hydrology (NZ), 25(1), 1-17.
- Houghton, J. G. (1979), A model for orographic precipitation in the north-central Great Basin, Monthly Weather Review, 107(11), 1462-1475.
- Jost, G., M. Weiler, D. R. Gluns, and Y. Alila (2007), The influence of forest and topography on snow accumulation and melt at the watershed-scale, Journal of Hydrology, 347(1), 101-115.
- Kerr, T., M. Clark, J. Hendrikx, and B. Anderson (2013), Snow distribution in a steep mid-latitude alpine catchment, Advances in Water Resources, 55(0), 17-24.
- Lundquist, J. D., S. E. Dickerson-Lange, J. A. Lutz, and N. C. Cristea (2013), Lower forest density enhances snow retention in regions with warmer winters: A global framework developed from plot-scale observations and modeling, Water Resources Research, 49(10), 6356-6370.
- Meromy, L., N. P. Molotch, T. E. Link, S. R. Fassnacht, and R. Rice (2012), Subgrid variability of snow water equivalent at operational snow stations in the western USA, Hydrological Processes.
- Molotch, N. P., and R. C. Bales (2005), Scaling snow observations from the point to the grid element: Implications for observation network design, Water resources research, 41(11), W11421.
- Schirmer, M., V. Wirz, A. Clifton, and M. Lehning (2011), Persistence in intra‐annual snow depth distribution: 1. Measurements and topographic control, Water Resources Research, 47(9).
- Scipión, D. E., R. Mott, M. Lehning, M. Schneebeli, and A. Berne (2013), Seasonal small-scale spatial variability in alpine snowfall and snow accumulation, Water Resources Research, 49(3), 1446-1457.
- Shook, K., and D. M. Gray (1998), Small‐scale spatial structure of shallow snowcovers, Hydrological processes, 10(10), 1283-1292.
- Tarboton, D., G. Bloschl, K. Cooley, R. Kirnbauer, and C. Luce (2000), Spatial Snow Cover Processes at Kuhtai and Reynolds Creek, in Spatial Patterns in Catchment Hydrology: Observations and Modelling., edited by G. Bloschl and R. Grayson, pp. 158-186, Cabridge University Press, Cambridge.
- Trujillo, E., J. A. Ramirez, and K. J. Elder (2007), Topographic, meteorologic, and canopy controls on the scaling characteristics of the spatial distribution of snow depth fields, Water Resources Research, 43(7).
- Trujillo, E., J. A. Ramírez, and K. J. Elder (2009), Scaling properties and spatial organization of snow depth fields in sub‐alpine forest and alpine tundra, Hydrological Processes, 23(11), 1575-1590.
- Varhola, A., N. C. Coops, M. Weiler, and R. D. Moore (2010), Forest canopy effects on snow accumulation and ablation: An integrative review of empirical results, Journal of Hydrology, 392(3–4), 219-233.
- Watson, F. G. R., T. N. Anderson, W. B. Newman, S. E. Alexander, and R. A. Garrott (2006), Optimal sampling schemes for estimating mean snow water equivalents in stratified heterogeneous landscapes, Journal of Hydrology The ICWRER – Symposium in Dresden, Germany, 328(3–4), 432-452.
The instructions below describe instructional activities, and field and data analysis steps to answer the following research questions:
- What is the volume of water stored in the DCEW snowpack in mid-March, 2014?
- How does snow water storage relate to cumulative winter precipitation?
- How is snow distributed in the watershed?
- What terrain properties control the distribution of snow?
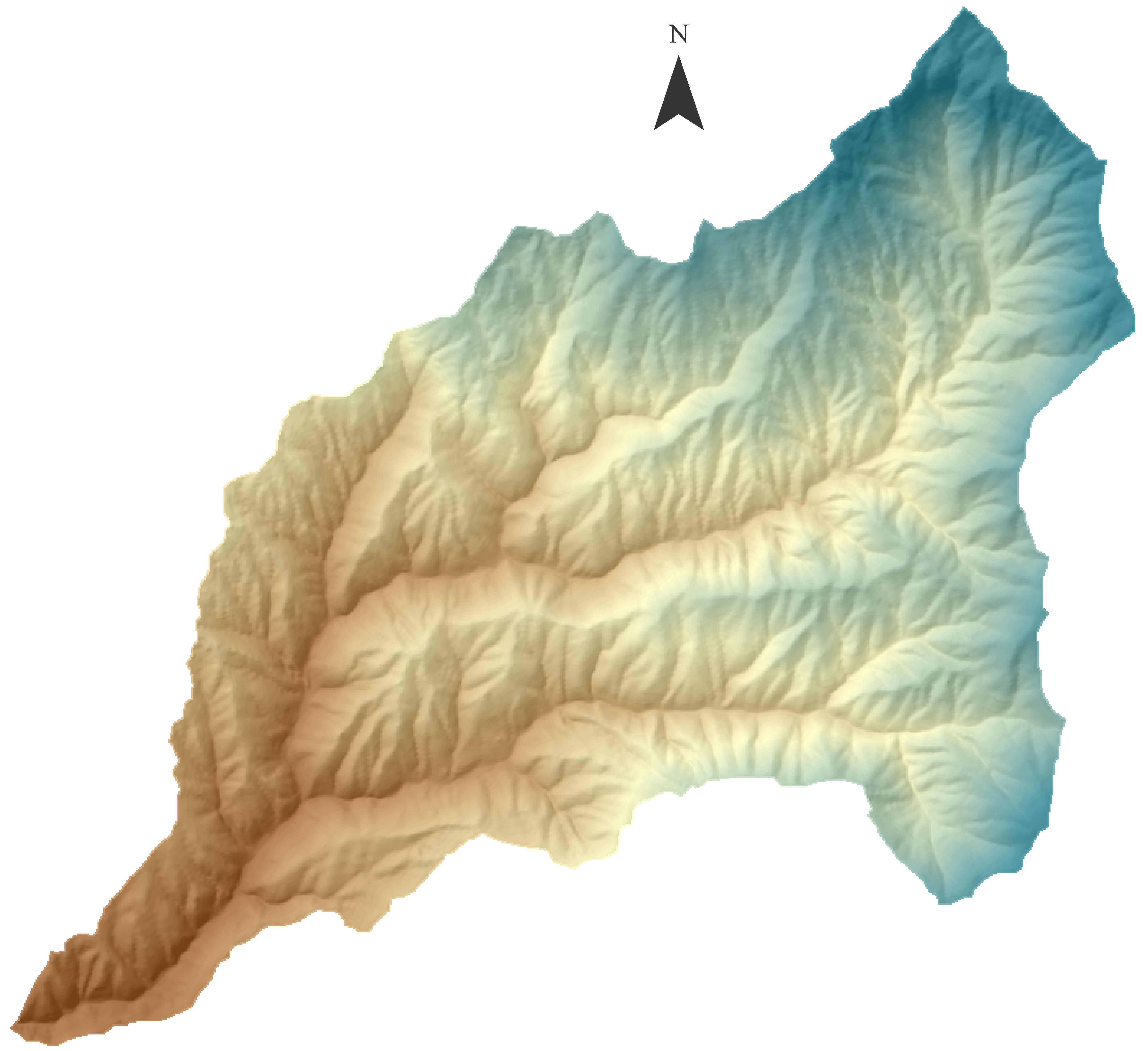
Project Files
- snowcamp_introduction.pptx
- Field data sheets
- Topographic maps
Activities
Sunday March 16
07:00 pm Social reception at the Bardenay Restaurant
The Bardenay is located at 610 W Grove Street a block north and a block east of the Grove hotel: Map
Monday March 17
08:30 Transport to Boise State University Department of Geosciences
Vans will pick participants up at the Grove Hotel in Downtown Boise for transport to Boise State University.
9:00 Introductions and Discussions (BSU ERB 4th floor commons)
- Welcome remarks: Jim McNamara
- WCWAVE Introduction: Lejo Flores
- Snow Camp Objectives and Logistics: Jim McNamara
- Snow Hydrology Introduction: Jim McNamara
- Snow Measurements: HP Marshall
9:30 Snow Camp Project Introduction (BSU ERB 4th floor commons)
(McNamara) The goal of this exercise is to estimate the volume and spatial distribution of water stored as snow in the DCEW. We will design an efficient field campaign to measure snow water equivalent throughout the watershed in one day. To design the field campaign participants will
- Determine the extent of the snow covered area using satellite imagery
- Determine the distribution of, and access to, representative snow units (RSU) using GIS
- Select sites within RSUs that can be visited by teams traveling by boot, snowshoe, ski, or snowmobile
- Decide what measurements to take in order to capture the local variability at each site
Research Questions:
In addition to our primary goal, we will address the following specific research questions
- How does snow water storage in the DCEW relate to cumulative precipitation at the meteorological stations?
- How is snow distribution related to terrain properties including elevation, aspect, and canopy cover?
10:00 Transport to Dry Creek Experimental Watershed
Vans will transport participants along the edge of DCEW towards Bogus Basin Ski Resort. Participants will pack a lunch to be eaten in the field. Vans will stop periodically to take a visual tour of the watershed before stopping at the Lower Deer Point station. Participants should be prepared to hike with snowshoes and work in the snow.
11:00 – 16:00 Introduction to Basic Field measurements
Participants travel in vans to Lower Deer Point meteorological station, travel 200 yards by snowshoe or ski to reach the site.
At the site, faculty will
- Demonstrate basic methods to measure snow depth, density, and snow water equivalent,
- Illustrate techniques for describing snow pit profiles, and
- Demonstrate new techniques for snow measurements using Ground Penetrating Radar
Participants will measure depth, density and SWE in transects approximately 200 m long traversing the site in order to illustrate the effect of various terrain properties on snow variability. Data will be analyzed in the evening while planning the basin-wide snow survey.
- Select 3-5 transects near the LDP site. One transect should traverse open and closed canopy areas, a second transect should traverse north facing and south facing aspects, a third transect should traverse wind exposed and protected areas
- Record the beginning and ending coordinates of each transect using a GPS
- Measure the depth (cm) of snow every 1m along the transect
- Measure the snow water equivalent (cm) using a federal sampler every 10m
- At each point, record qualitative estimates of aspect, canopy density, and wind exposure
- Select random locations near LDP
- Collect 50 snow depth measurements at random locations within a 10 m radius
Lunch: Snack lunches will be eaten during field exercise.
16:00 Pioneer Condominiums at Bogus Basin
Vans transport participants to the Pioneer Condominiums for check in.
18:00 Dinner Cooked at Residence
Planning in progress
Evening Data Analysis and Campaign Planning
The goal of the evening activity is to plan an efficient sampling campaign to estimate the volume of water held as snow in the DCEW. First, participants will analyze field data collected at the LDP in order to understand local sources of variability. Second, participants will evaluate the terrain in the DCEW to select sampling locations.
LDP Snow Variability
- Compile transect survey data into spreadsheets
- Compute the density for each SWE measurement location
- Compute mean and standard deviation for snow depth, snow density, and snow water equivalent
- Construct variograms for each transect to determine correlation lengths (optional)
- Qualitatively assess relationships between snow and terrain properties. Participants will compute correlation coefficients if we have quantitative measures of terrain properties.
- Analysis questions:
- What is the relative importance of depth and density on the variability of SWE?
- What terrain properties control spatial variability of SWE?
- What terrain properties did we neglect?
Field Campaign Planning
Our goal is to estimate the volume of water stored as snow in the DCEW. We will work in three independent teams in order to assess the reproducibility of our estimates. Each team will design a field campaign that captures the sources of variability SWE in the DCEW while maintaining field efficiency. Final presentations will occur on Wednesday morning wherein each team will present their conclusions, defend their methods, and discuss implications for hydrologic modeling.
Important questions to incorporate into the sampling program include
- What is extent the snow covered area?
- Where are the access points to the watershed?
- What are the abilities and limitations of the group?
- How many stations can be visited?
- Where should we sample?
- What should we measure at each sampling site (how many depth and SWE measurements)?
GIS coverages for the DCEW are available here: earth.boisestate.edu/drycreek/data/spatial-data. GIS software will be available on laptop computers.
Tuesday March 18
7:30 Breakfast
Planning in progress
8:30-16:00 Field Exercise
Each will implement their designed field campaign. Participants should be prepared to spend at least 7 hours outside in the snow collecting and recording data. Sack lunch supplies will be provided. See What to Bring for a list of required and recommended supplies.
16:30 Free time
Participants will return from the field exercise no later than 4:30.
18:00 Dinner
Planning in progress
Evening Data Analysis
Teams will compile and analyze field data to estimate the volume of water stored as snow, as well as answer research questions posed above.
Volume of water stored as snow
Issues to consider include:
- How to average depth and SWE measurements at each field site?
- How to average site measurements across the watershed? Direct average or weighted average by representative snow units?
Relationship between cumulative winter precipitation and SWE on the ground
- From the Dry Creek data access page (earth.boisestate.edu/drycreek), compile meteorological data for Lower Weather, Treeline, and Boise Ridge meteorological stations from December 15, 2013 through the current date.
- For each station, determine the depth of precipitation that fell as snow by analyzing precipitation, air temperature, and other relevant meteorological variables.
- Determine the precipitation lapse rate by plotting total precipitation from step 2 against elevation.
- Using data from field surveys, construct a plot of SWE on the ground against elevation.
- Discuss differences between precipitation lapse rate and SWE lapse rate.
Relationships between SWE distribution and terrain properties
- Compute correlation coefficients between site average SWE and terrain properties including elevation, aspect, and canopy density. Discuss results.
Wednesday March 19
8:00 Breakfast
Planning in progress
9:00 Project Presentations
Planning in progress
10:00 Terrain Exploration Free Time
Options include:
- Downhill ski at Bogus Basin Resort (lift tickets and equipment rental not included in cost of camp)
- Nordic ski or snowshoe at Bogus Basin Resort (lift tickets and equipment rental not included in cost of camp)
- Visit CryoGARS research site within Bogus Basin (lift ticket required)
- Hike, relax, etc…
Late Afternoon Return to Boise
Vans will depart for Boise at various times throughout the afternoon to accommodate group needs.