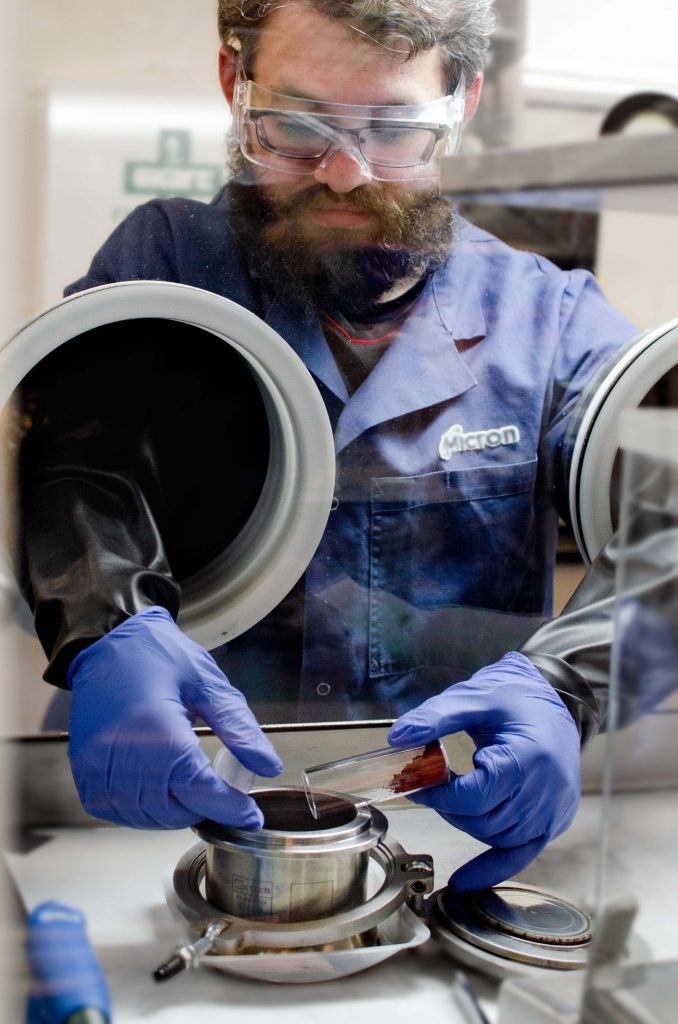
Sam is a third-year Ph.D. student in the Micron School of Materials Science & Engineering. He earned his B.S. in Materials Science & Engineering from Purdue and then worked at Boeing in polymer composite research & development. After working at Boeing for a few years, he wanted to return to fundamental materials science research, so he came to Boise State for graduate school. In Sam’s first year here, he developed novel mechanical alloying techniques for thermoelectric materials processing. His work titled “Novel Synthesis and Processing Effects on the Figure of Merit for NbCoSn, NbFeSb and ZrNiSn based half-Heusler Thermoelectrics” was recently accepted for publication in the Journal of Solid State Chemistry.
In early 2019, Sam was awarded a College of Engineering Seed Grant ($10K) to explore efficient methods for synthesizing black phosphorus. Black phosphorus is a tunable 2D material that shows promise in optoelectronic applications. The current production process is expensive and involves applying high pressure to white phosphorus. Sam developed a more economical method to produce black phosphorus by high-energy ball-milling red phosphorus, an inexpensive by-product of the fertilizer industry.
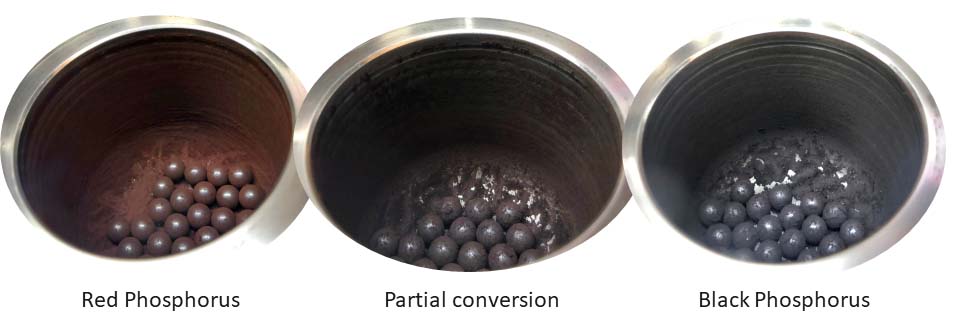
Sam’s work showed so much promise, the Advanced Materials Lab was recently granted two awards to continue the work in conjunction with the Advanced Nanomaterials & Manufacturing Lab and Iris Light Technologies:
- DoD-AFWERX STTR Phase 1 ($75K) to produce black phosphorus inks and print silicon photonic lasers.
- IGEM Commerce Award ($242K) to scale the ball-milling process up to a commercially-viable milling process.
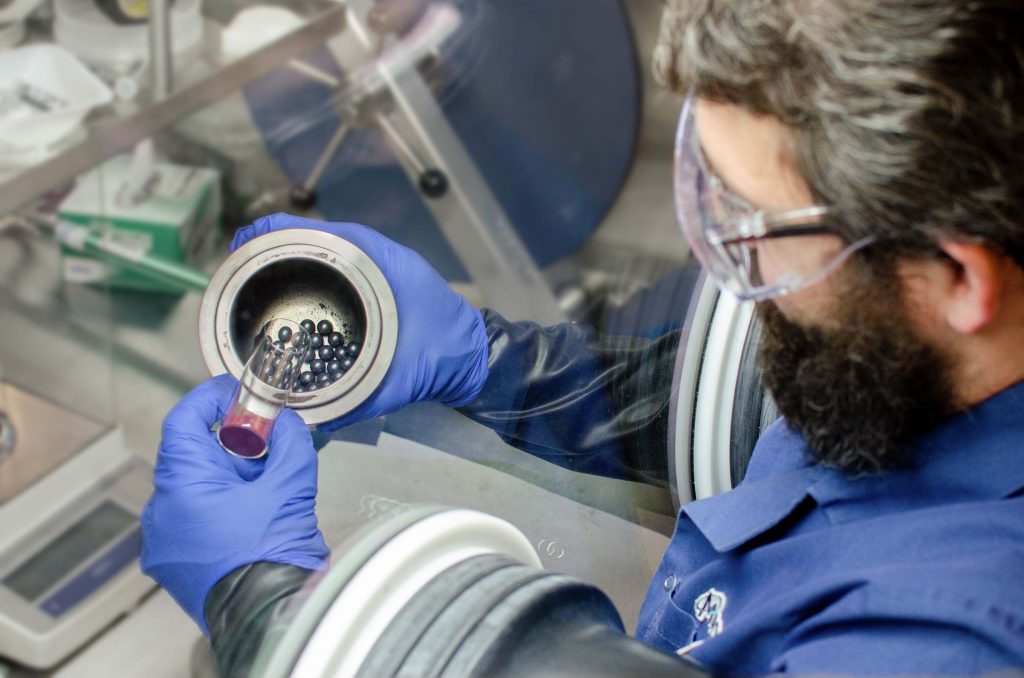
In a partnership with Argonne National Lab, the AML and Iris Light Technologies have also submitted for a DOE Technology Commercialization Fund to study AI-guided synthesis of black phosphorus for printing lasers on silicon photonic chips.Sam is presenting his research on the mechanochemical synthesis of black phosphorous at the 2020 Materials Research Society Meeting in Phoenix this April. The abstract for his presentation is below.
With the promise of novel optoelectronic devices using black phosphorus (BP), there is a need for a large-scale synthesis method for BP compatible with on-chip integration. Herein, we attain large scale mechanochemical conversion of red phosphorus (RP) to black phosphorus (BP) via high energy ball milling. During the milling process, the collisions of hardened steel media rapidly compress amorphous RP into crystalline, orthorhombic BP flakes, with ac conversion yield >95% for up to 5g of bulk BP powder. Commercial scale-up of BP production can be extrapolated by empirically derived conversion kinetics from lab scale, high energy ball mills. RP’s milling conversion kinetics, as monitored via ex situ XRD, shows a sigmoidal behavior best described by the Avrami rate model. For <60% conversion, BP is rapidly formed at the expense of RP following an autocatalytic behavior with induction times proportional to the milling intensity. At ≥60% conversion, milling conversion rate suddenly decreases, indicative of a growth mechanism change with presumably a higher activation energy, thereby requiring higher impact energies for complete conversion. Further, by comparing the specific milling dose, namely, the energy input required for the phase transformation to reach completion, versus the conversion progress, we can determine the optimum milling conditions. We therefore ascertain the BP specific milling dose (50 kJ/g) and minimum impact energy (25 mJ/impact), both necessary to scale up production and minimize the power consumed in a tumbler milling vessel. Finally, the as-synthesized BP powder exfoliates more readily than single crystal BP sources in solution, as revealed by concentrations obtained from UV-Vis absorption under the same exfoliation conditions. The milled, converted BP solution is roughly 6-fold the concentration of the single crystal solution. High energy ball milling is demonstrated as a large scale, viable synthesis route for BP.